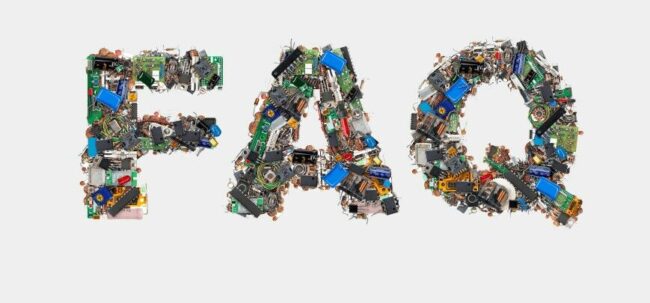
What are the basic components of a differential transformer?
A differential transformer, also known as a linear variable differential transformer (LVDT), is a type of sensor used to measure linear displacement or position. It operates on the principle of electromagnetic induction and consists of several essential components that enable its function. The main components of a differential transformer are:
- Primary Coil: The primary coil is the input coil through which an alternating current (AC) is passed. When AC current flows through the primary coil, it generates a varying magnetic field around it.
- Secondary Coils: There are two secondary coils wound symmetrically around the primary coil. These secondary coils are connected in series-opposing configuration. The changing magnetic field induced by the primary coil’s current induces voltages in the secondary coils.
- Core Assembly: The core assembly consists of a magnetic core that can move linearly within the assembly. This core is often connected to the object whose displacement or position is being measured. The core’s movement within the coil assembly affects the magnetic coupling between the primary and secondary coils.
- Housing: The primary and secondary coils, as well as the core assembly, are enclosed within a housing that provides protection from environmental factors and mechanical damage.
- Signal Conditioning Circuitry: The output voltage from the secondary coils is usually very small and needs to be amplified and conditioned for accurate measurement. Signal conditioning circuitry, including amplifiers and filters, is used to process and improve the quality of the output signal.
- Output Terminals: The output terminals of the secondary coils are connected to the signal conditioning circuitry. The voltage difference between the two secondary coils is measured to determine the displacement or position.
In operation, when the core moves within the coil assembly due to linear displacement, it changes the magnetic coupling between the primary and secondary coils. This change in magnetic coupling induces different voltages in the secondary coils, resulting in a differential voltage output that corresponds to the object’s displacement. This differential output voltage is processed by the signal conditioning circuitry to provide accurate displacement or position measurement.
Differential transformers are commonly used in applications where precise linear position sensing is required, such as industrial automation, robotics, aerospace, and more. Their reliability, accuracy, and durability make them suitable for various challenging environments and measurement scenarios.
A part that is applicable to both a contact IC card and a non-contact IC card:
One component that is applicable to both contact and non-contact IC cards is the integrated circuit chip (IC chip). The IC chip is the core component of the card’s functionality, containing the microprocessor and memory necessary to process data and perform various functions. The IC chip is responsible for storing and processing information, executing security protocols, and enabling communication between the card and external devices.
In contact IC cards, the IC chip is embedded within the card’s physical body and is connected to the card’s contacts, which are typically exposed on the surface of the card. When the card is inserted into a card reader or terminal, the contacts establish an electrical connection with the reader, allowing data exchange.
In non-contact IC cards, also known as RFID (Radio-Frequency Identification) cards, the IC chip is typically integrated into the card’s structure and connected to an antenna. The chip and antenna together form a passive or active RF communication system that enables wireless communication between the card and a reader or terminal. When the card is brought close to the reader, electromagnetic energy from the reader powers the chip, allowing it to transmit data back to the reader using radio waves.
The IC chip in both contact and non-contact IC cards can store various types of information, such as user data, authentication keys, account information, and more. It plays a pivotal role in ensuring the functionality, security, and integrity of the card’s operations, regardless of whether it is used in a contact or non-contact mode.
What is the role of the rectifier?
A rectifier is an electrical device that converts alternating current (AC), which periodically reverses direction, into direct current (DC), which flows in only one direction. The process of converting AC to DC is called rectification. Rectifiers play a crucial role in various electronic and electrical applications. Here’s a breakdown of the role of a rectifier:
- Power Supply Conversion: Most of the electronic devices we use, such as computers, smartphones, and televisions, operate on DC. However, the electricity supplied to homes and industries is AC. Power adapters and chargers for these devices have built-in rectifiers that convert the AC from the mains into the DC required by the electronic device.
- Battery Charging: When charging batteries, a DC current is required. In battery chargers, rectifiers help convert the AC supply into DC to facilitate this charging process.
- Welding: In electric welding machines, rectifiers convert AC power from the mains to DC, as most welding processes require direct current due to its steady nature and better heat control.
- Electroplating: The electroplating process, which deposits one metal onto another by using an electric current, requires a DC supply. Rectifiers are used to provide this direct current.
- Signal Demodulation: In old analog radio receivers, rectifiers (often in the form of diodes) were used to demodulate amplitude-modulated (AM) signals to extract the original audio signal.
- Voltage Multipliers: Rectifiers, in combination with capacitors, can be used to create voltage multipliers that produce higher DC voltages than the peak input AC voltage.
- Conversion for HVDC (High-Voltage Direct Current): For long-distance power transmission, HVDC systems are used because they have lower losses compared to AC transmission. Rectifiers are used to convert high-voltage AC to DC at the transmitting end, and inverters (which can be thought of as the opposite of rectifiers) are used to convert it back to AC at the receiving end.
Rectifiers can be of different types based on their construction and output characteristics:
- Half-Wave Rectifier: Uses a single diode and rectifies only half of the AC waveform.
- Full-Wave Rectifier: Uses two diodes in a center-tapped transformer setup or four diodes in a bridge configuration to rectify the entire AC waveform.
- Bridge Rectifier: Uses four diodes in a specific configuration to achieve full-wave rectification without the need for a center-tapped transformer.
In summary, the primary role of a rectifier is to convert AC into DC to cater to various electrical and electronic applications where a steady and unidirectional voltage and current are required.
What is superframe routing?
“Superframe routing” doesn’t appear to be a standard or widely recognized term in the context of networking or technology. It’s possible that there might be a misunderstanding or confusion with the terminology.
If you’re referring to routing concepts in networking, here are a few relevant terms that might be more aligned with common networking terminology:
- Routing: Routing is the process of determining the optimal path for data to travel from the source to the destination in a network. Routers use routing tables and algorithms to make decisions about how to forward data packets.
- Superframe: In some networking contexts, a “superframe” could refer to a larger unit of time or data that encapsulates multiple frames. For example, in the context of wireless networks like Zigbee, a superframe is a structured time period that contains a specific sequence of active and inactive periods for efficient communication.
- Routing Protocol: A routing protocol is a set of rules and conventions used by routers to communicate and exchange information about network topology. Common routing protocols include OSPF (Open Shortest Path First), BGP (Border Gateway Protocol), and RIP (Routing Information Protocol).
- Route: A route is a specific path that data takes through a network from source to destination. Routing involves determining and maintaining these routes.
- Routing Table: A routing table is a data structure used by routers to store information about routes, including destination addresses, next-hop routers, and interface information.
- Static Routing: In static routing, administrators manually configure the routing table on routers. Routes do not change automatically in response to network changes.
- Dynamic Routing: Dynamic routing protocols allow routers to exchange information and adapt to network changes automatically. Examples include EIGRP (Enhanced Interior Gateway Routing Protocol) and IS-IS (Intermediate System to Intermediate System).
- Routing Metrics: Routing metrics are values used by routers to make decisions about the best path to forward data. Metrics can include factors like bandwidth, latency, and hop count.
If you have a specific context or technology in mind, please provide more details, and I’d be happy to provide information relevant to that context.
What are the four types of linear induction synchronizers?
The term “linear induction synchronizers” doesn’t appear to be a widely recognized term or concept in the context of technology or engineering. It’s possible there might be some confusion or a misunderstanding of terminology.
If you are referring to linear induction motors or similar concepts, here are a few relevant terms that might be closer to what you’re looking for:
- Linear Induction Motor (LIM): A linear induction motor is a type of motor that produces motion in a linear direction, as opposed to the rotational motion of traditional motors. It works on the principle of electromagnetic induction, where alternating current (AC) in the primary winding induces currents in the secondary conductor, creating a magnetic field that propels the conductor along a track.
- Linear Synchronous Motor (LSM): A linear synchronous motor is another type of linear motor that operates based on electromagnetic principles. Unlike a linear induction motor, an LSM operates synchronously with the AC power supply frequency. It requires position feedback to maintain synchronization between the stator and the moving part.
- Maglev (Magnetic Levitation) Systems: Maglev systems use magnetic fields to levitate and propel vehicles, typically trains, along a guideway. These systems often utilize linear induction or linear synchronous motor principles to achieve propulsion without direct physical contact with the track.
- Linear Induction Generator (LIG): A linear induction generator is a device that converts mechanical energy into electrical energy using the principles of electromagnetic induction. It’s the reverse of a linear induction motor: instead of applying a current to induce motion, motion is applied to induce a current.
It’s worth noting that the terminology can vary depending on the specific field or industry you’re referring to, so providing additional context could help clarify the concept you’re asking about. If you have a specific context in mind, please provide more details, and I’d be happy to assist further.
What is the node method?
The “node method” is a technique used in electrical circuit analysis to solve for unknown voltages in a circuit. It’s also known as the “node-voltage method” or “modified nodal analysis.” The node method is particularly useful for analyzing circuits with multiple interconnected nodes (points where three or more circuit elements meet).
In the node method, the circuit is divided into nodes, which are points where the current can branch out. Each node is assigned a unique label or name. The basic steps of the node method are as follows:
- Choose a Reference Node: One node is selected as the reference node (usually the one with the most connections). The voltage at the reference node is usually taken as zero (ground).
- Assign Node Voltages: Assign a variable (voltage) to each of the other nodes. These variables are often represented as “V1,” “V2,” and so on.
- Apply Kirchhoff’s Current Law (KCL): At each non-reference node, apply KCL to write an equation that relates the currents entering and leaving the node. These equations are based on Ohm’s law and the relationships between voltage, current, and resistance (or impedance).
- Write Equations: Write equations for each non-reference node using KCL. These equations will be in terms of the assigned node voltages.
- Use Ohm’s Law: Substitute the node voltages into the KCL equations using Ohm’s law to represent currents in terms of node voltages.
- Solve Simultaneous Equations: The resulting equations form a system of simultaneous equations. Solve this system of equations to find the node voltages.
- Calculate Other Parameters: Once the node voltages are known, you can use them to calculate other circuit parameters such as currents, power, and voltage drops across components.
The node method is especially useful for analyzing complex circuits with multiple voltage sources, dependent sources, and resistors. It’s a systematic way of solving circuit problems and obtaining voltage values at various nodes within the circuit.
It’s important to note that while the node method is powerful, it might require solving a system of equations, which can be time-consuming for larger circuits. In such cases, computer-based circuit analysis tools can be used to automate the process.
What is the rater?
Also known as “magnification Resistors.”A Resistors used to increase the meter range in voltage measurement.Used in series with the voltmeter, the measured voltage value should be equal to the voltmeter reading multiplied by a multiple.
What level of coordination should be noted for the application of non-open RFID systems?
In the context of non-open RFID systems, coordination is crucial to ensure the proper functioning and integration of the RFID technology within a specific application or environment. Non-open RFID systems typically refer to proprietary or closed systems that are developed and maintained by specific vendors or organizations. The level of coordination required for the application of non-open RFID systems depends on several factors:
- Interoperability: If you are using non-open RFID systems from different vendors or sources, coordination is essential to ensure that these systems can work together seamlessly. This might involve coordinating protocols, data formats, communication frequencies, and software interfaces.
- System Integration: Integrating RFID systems with existing infrastructure and software requires coordination to ensure compatibility and minimal disruption to existing processes. This might involve coordinating with IT departments and system administrators.
- Hardware and Software Compatibility: Coordination is needed to ensure that the hardware components (RFID readers, tags, antennas) and software applications used in the non-open RFID system are compatible with each other and meet the specific requirements of the application.
- Security: Non-open RFID systems often involve proprietary security protocols. Coordinating security measures, such as encryption, authentication, and access control, is critical to protecting sensitive data and preventing unauthorized access.
- Vendor Support: Since non-open RFID systems are often vendor-specific, coordination with the vendor is important for installation, configuration, troubleshooting, and ongoing support.
- Deployment Strategy: Coordinating the deployment strategy involves planning the physical installation of RFID readers, antennas, and tags to optimize performance and coverage.
- Testing and Validation: Comprehensive testing and validation should be coordinated to ensure that the non-open RFID system meets the desired performance, accuracy, and reliability standards.
- Training: Training personnel on how to use and maintain the non-open RFID system effectively requires coordination to ensure that everyone involved understands its capabilities and operation.
- Regulatory Compliance: Coordination with regulatory authorities, if applicable, is essential to ensure that the non-open RFID system complies with relevant standards and regulations.
- Data Management: Coordination of data management processes, including data collection, storage, analysis, and sharing, is crucial to derive insights and value from the RFID system.
Overall, the level of coordination required for the application of non-open RFID systems is comprehensive and involves various aspects of technology integration, system design, deployment, and maintenance. Collaboration between relevant stakeholders, including vendors, IT personnel, end-users, and management, is key to a successful implementation.
What is the capacitance of the capacitor?
The capacitance of a capacitor is a measure of its ability to store electrical charge when a voltage difference (potential difference) exists between its two plates. It’s a fundamental property of a capacitor and is represented by the symbol “C.” Capacitance is measured in units called farads (F), named after the physicist Michael Faraday.
Mathematically, the capacitance of a capacitor is defined as the ratio of the magnitude of the stored charge (Q) on one of its plates to the potential difference (V) across the plates:
C=Q/V
Where:
- C represents the capacitance in farads (F).
- Q represents the charge stored on one of the plates in coulombs (C).
- V represents the potential difference between the plates in volts (V).
The larger the capacitance value, the more charge a capacitor can store for a given potential difference. Capacitance is a property that depends on the physical characteristics of the capacitor, such as the area of the plates, the distance between them (dielectric thickness), and the properties of the dielectric material between the plates.
Common units for capacitance include:
- Microfarad (μF) = 10−610−6 farads
- Nanofarad (nF) = 10−910−9 farads
- Picofarad (pF) = 10−1210−12 farads
Capacitors are used in various electronic circuits for energy storage, filtering, timing, and signal coupling. They play a crucial role in many electronic devices and systems.
Briefly describe the level of signaling control?
No Signaling: In this most basic case, the node has no information about the neighbor nodes.The node will decide to send the packet without knowing if the neighbor node exists.Full signaling: Under full signaling, the node not only periodically transmits beacons to discover neighbor nodes, but also exchanges information with neighbor nodes about which data packets or encoded data packets are stored locally, that is, the sequence of data packets.Number or encoding vector of the encoded packet.
COMMENTS