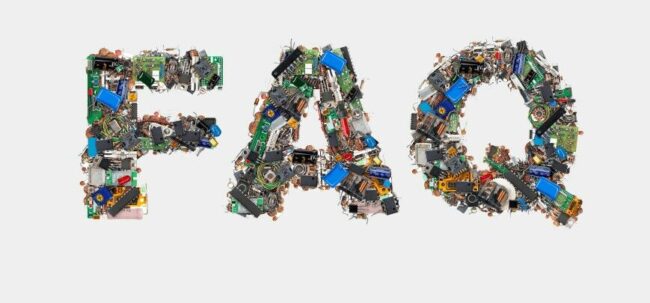
What are the general driving methods?
General driving methods refer to the fundamental techniques and practices that individuals use when operating a motor vehicle safely and responsibly. These methods are crucial for ensuring the safety of the driver, passengers, pedestrians, and other road users. Here are some general driving methods:
- Observation and Awareness:
- Always be attentive and aware of your surroundings.
- Regularly check your mirrors to monitor traffic behind and beside you.
- Scan the road ahead for potential hazards, such as other vehicles, pedestrians, and road signs.
- Speed Management:
- Obey posted speed limits and adjust your speed according to road and weather conditions.
- Reduce speed in adverse weather (rain, snow, fog) and on slippery roads.
- Following Distance:
- Maintain a safe following distance between your vehicle and the one in front of you.
- The “3-second rule” is a guideline for following distance under normal conditions.
- Lane Discipline:
- Stay in your lane and use turn signals when changing lanes.
- Follow proper lane etiquette, such as keeping to the right except when passing on multi-lane roads.
- Signal and Communication:
- Use turn signals to indicate your intentions to other drivers.
- Use headlights, brake lights, and horn to communicate effectively.
- Defensive Driving:
- Anticipate the actions of other drivers and be prepared for unexpected maneuvers.
- Avoid aggressive driving behaviors like tailgating and road rage.
- Stopping and Braking:
- Use brakes smoothly and avoid sudden, hard braking.
- Maintain a safe stopping distance from vehicles in front of you.
- Right of Way:
- Yield the right of way as required by traffic laws and road signs.
- Never assume other drivers will yield when they should.
- Parking and Maneuvering:
- Park legally and considerately, following local parking regulations.
- Practice safe maneuvers when reversing or parallel parking.
- Stay Sober and Alert:
- Never drive under the influence of alcohol, drugs, or medications that impair your abilities.
- Avoid driving when fatigued, as it can affect your reaction time and judgment.
- Use of Safety Equipment:
- Always wear seatbelts, and ensure all passengers do the same.
- Use child safety seats for young passengers as appropriate.
- Adapt to Road Conditions:
- Adjust your driving to suit the road conditions, such as rain, ice, or heavy traffic.
- Use headlights during low visibility conditions.
- Obey Traffic Signs and Signals:
- Follow all traffic signs, signals, and road markings.
- Be aware of and obey speed limits, stop signs, yield signs, and other regulatory signs.
- Stay Informed:
- Keep up to date with changes in traffic laws and regulations.
- Stay informed about road closures, construction zones, and detours.
- Emergency Procedures:
- Know how to respond to emergencies, such as vehicle breakdowns and accidents.
- Pull over and use hazard lights if your vehicle experiences problems.
Remember that safe driving is not only about following rules but also about making responsible choices that prioritize the safety of everyone on the road. Defensive driving, courtesy, and patience are essential components of being a safe and responsible driver.
What is the relationship between LTE and 4G?
LTE (Long-Term Evolution) and 4G (4th Generation) are related in that LTE is a key technology that is often used to provide 4G wireless communication. Here’s the relationship between LTE and 4G:
- 4G Definition:
- 4G is a generation of wireless communication technology that succeeded 3G (3rd Generation) networks.
- It was developed to provide faster data speeds, lower latency, and improved overall performance compared to 3G.
- LTE as a Part of 4G:
- LTE, which stands for Long-Term Evolution, is a specific technology standard within the 4G family.
- LTE is the primary air interface or wireless communication standard used to deliver high-speed data and multimedia content in 4G networks.
- Data Speeds and Performance:
- LTE significantly enhances data speeds compared to 3G, making it a key enabler of the higher data rates associated with 4G.
- 4G networks, including those utilizing LTE, are designed to offer peak data rates that can be several times faster than typical 3G networks.
- Advanced Features:
- 4G networks, including LTE, introduce advanced features like low-latency communication, which is important for real-time applications like online gaming and video conferencing.
- LTE also supports higher-quality voice calls through technologies like Voice over LTE (VoLTE).
- Global Standards:
- LTE is part of the global standards set by the 3rd Generation Partnership Project (3GPP) for 4G wireless technology.
- 4G networks can use different frequency bands and technologies depending on the region and carrier, but LTE is a common technology used in many 4G deployments worldwide.
In summary, LTE is a specific technology standard that is commonly used within 4G networks to provide high-speed data communication and other advanced features. When you hear about 4G networks, they often rely on LTE as a key component to deliver the performance and capabilities associated with the 4th generation of wireless communication technology.
What are the possible software errors when the SF LED is lit?
The “SF LED” typically refers to the “Status Fail” LED on networking equipment or devices like routers, switches, or network appliances. When the SF LED is lit, it indicates that there may be an issue or error with the device’s status or operation. There are several possible software-related errors or issues that can cause the SF LED to be lit, including:
- Software Crash or Hang: If the device’s operating system or software encounters an error or becomes unresponsive, it may trigger the SF LED to indicate a failure in the software. This could be due to a bug, a memory issue, or other software-related problems.
- Configuration Errors: Misconfigured settings or parameters in the device’s software can lead to operational problems. For example, if there are incorrect network settings, routing configurations, or access control lists, it may trigger the SF LED.
- Firmware Issues: Firmware is the software that controls the hardware components of the device. If there’s a problem with the firmware, such as a corrupted firmware update or an outdated version, it can lead to a status fail condition.
- Software Update Problems: Updating the device’s software or firmware can sometimes introduce errors if the update process fails or if there are compatibility issues between the new software version and the hardware.
- Resource Exhaustion: If the device’s software consumes all available system resources (CPU, memory, disk space), it can result in a status fail condition. This often happens due to memory leaks or excessive resource usage by certain processes.
- Security Vulnerabilities: Exploitable security vulnerabilities in the device’s software can lead to unauthorized access or malicious activity, triggering the SF LED to indicate a security-related failure.
- Log and Diagnostic Errors: Devices typically maintain logs and diagnostic information. If there are errors or warnings logged by the software, they can lead to the activation of the SF LED.
- Software Dependencies: Sometimes, the software relies on external services or dependencies. If those services fail or become unavailable, it can cause a status fail condition.
- Hardware-Software Interaction: In some cases, hardware problems can manifest as software errors. Faulty hardware components can lead to software-related failures.
To troubleshoot and resolve issues when the SF LED is lit, it’s essential to investigate logs, perform diagnostics, and check the device’s configuration and software/firmware versions. Depending on the specific device and situation, you may need to engage technical support or follow prescribed troubleshooting procedures provided by the manufacturer.
What are the basic characteristics of SJAl000?
(1) The CAN controller independent of the PCA82C200 is pin compatible.
(2) Electrically compatible with the CAN controller independent of the PCA82C200.
(3) It has the PCA82C200 mode (ie the default BasicCAN mode).
(4) Extended receive buffer (64 bytes, first in first out FIFO).
What is the analog output type liquid level sensor used for testing?
Analog output type liquid level sensors are commonly used for testing and monitoring liquid levels in various applications. These sensors provide a continuous analog voltage or current signal that corresponds to the liquid level being measured. The choice of an analog output sensor for testing depends on the specific requirements of the application. Here are some common uses of analog output liquid level sensors for testing:
- Tank Level Monitoring: Analog level sensors are frequently used in tanks and reservoirs to monitor the level of liquids, such as water, chemicals, or fuel. This is valuable in industrial processes, agriculture, and environmental monitoring.
- Industrial Process Control: Analog liquid level sensors are used in industrial settings to control and optimize processes. For example, in manufacturing, they can be used to ensure a consistent supply of liquid to a process.
- Liquid Storage and Inventory Management: Businesses that store and distribute liquids, such as fuel depots or chemical storage facilities, use analog sensors to keep track of inventory levels and trigger refills when necessary.
- Environmental Monitoring: Analog output liquid level sensors are used in environmental applications, such as monitoring water levels in rivers, lakes, and reservoirs, as well as in groundwater monitoring wells.
- Laboratory and Research: Analog level sensors are used in laboratories and research facilities for experiments that involve measuring and controlling liquid levels.
- Medical Devices: Some medical devices, such as infusion pumps and dialysis machines, use analog liquid level sensors to ensure accurate fluid delivery.
- HVAC Systems: Analog liquid level sensors are employed in heating, ventilation, and air conditioning (HVAC) systems to monitor and control the levels of coolant or refrigerant in systems.
- Agricultural Irrigation: Analog sensors are used in agricultural irrigation systems to monitor and control water levels in irrigation reservoirs and tanks.
- Automotive and Transportation: Analog liquid level sensors can be found in vehicles to measure fuel levels, coolant levels, and oil levels, helping to provide accurate readings to drivers.
- Food and Beverage Production: Analog sensors are used in food and beverage processing to monitor the levels of liquids, such as ingredients and mixing ratios.
Analog output liquid level sensors can use various output signals, such as voltage (e.g., 0-5V, 0-10V), current (e.g., 4-20mA), or resistance (e.g., potentiometric sensors). The choice of sensor type and signal depends on the specific requirements of the testing or monitoring application.
What are the components of ion selective electrodes?
Ion-selective electrodes (ISEs) are analytical instruments designed to measure the concentration of specific ions in a solution. These electrodes consist of several key components that enable their selective response to a particular ion of interest. The main components of ion-selective electrodes include:
- Ion-Selective Membrane (ISM): This is the heart of the ion-selective electrode. The ISM is a specialized membrane that selectively allows the passage of the ion you want to measure while excluding interference from other ions. The composition of the ISM varies depending on the target ion. For example, a pH electrode uses a glass membrane sensitive to hydrogen ions (H+), while other types of ISEs use organic polymer membranes for specific ions like sodium (Na+), potassium (K+), chloride (Cl-), or fluoride (F-).
- Reference Electrode: To measure the potential difference (voltage) generated by the selective response of the ISM to the ion of interest, the ISE needs a reference electrode. The reference electrode provides a stable, known potential against which the potential of the ISM is measured. Common reference electrodes used in ISEs include Ag/AgCl electrodes, calomel electrodes (Hg/Hg2Cl2), and others, depending on the specific requirements of the measurement.
- Inner Filling Solution: The reference electrode typically contains an inner filling solution that maintains the stability of the reference potential. This filling solution is in direct contact with the reference electrode’s internal element (e.g., Ag/AgCl). The composition of the filling solution can vary depending on the reference electrode design.
- Junction: Many ISEs have a junction, also known as a salt bridge, that allows for the exchange of ions between the sample solution and the inner reference electrode solution. The junction may consist of a porous ceramic, a liquid junction, or a solid-state junction, depending on the type of ISE.
- Connector and Cable: ISEs are typically equipped with connectors and cables that allow them to be connected to a pH meter or ion analyzer. These connectors ensure a secure electrical connection between the electrode and the measuring device.
- Housing: The electrode components are housed in a protective body made of materials such as glass, plastic, or epoxy. The housing provides mechanical protection to the sensitive components and helps maintain the stability of the electrode.
- Electrode Body: This is the part of the electrode that is immersed in the sample solution. It typically includes the ISM and the junction, which come into direct contact with the sample.
- Reference Electrode Junction: In some ISEs, there may be a separate junction associated with the reference electrode. This junction ensures that the reference electrode maintains electrical contact with the inner filling solution.
These components work together to enable the ion-selective electrode to measure the concentration of a specific ion in a solution by detecting the potential difference generated as a result of the ion’s selective interaction with the ion-selective membrane. Depending on the type of ion being measured and the specific electrode design, there can be variations in the construction and components of ion-selective electrodes.
What is active power?
Active power, also known as real power, is one of the fundamental concepts in electrical engineering and power systems. It represents the actual power consumed or utilized by electrical devices and systems to perform useful work, such as generating mechanical motion, heating, lighting, or computing. Active power is typically measured in watts (W) or kilowatts (kW) and is an essential parameter in electrical engineering and energy management.
Here are key points to understand about active power:
- Definition: Active power is the power that is continuously transferred from a power source (e.g., a generator or a utility grid) to a load (e.g., electrical appliances or machinery) to perform useful work. It is the rate at which energy is converted from electrical form to another form, such as mechanical, thermal, or electromagnetic energy.
- Mathematical Representation: Active power is often represented by the symbol “P” and is expressed in watts (W) or kilowatts (kW). Mathematically, it is given by the formula:P = V × I × cos(θ)where:
- P is the active power (in watts or kilowatts).
- V is the voltage (in volts).
- I is the current (in amperes).
- θ is the phase angle (the angle between voltage and current waveforms).
- Power Factor: The term “cos(θ)” in the formula represents the power factor. The power factor is a dimensionless number that ranges from 0 to 1, indicating how effectively electrical power is being converted to useful work. A power factor of 1 (cos(θ) = 1) indicates a purely resistive load, while a power factor less than 1 indicates a load with reactive components like inductance or capacitance.
- Units: Active power is measured in watts (W) or kilowatts (kW). For example, a light bulb might consume 60 watts of active power, while a large industrial motor might require 100 kilowatts.
- Billing and Energy Costs: In many electrical utility systems, consumers are billed for active power consumption. This is because active power represents the actual work being done and contributes to energy costs.
- Importance: Active power is crucial for sizing electrical equipment, designing power systems, and assessing the efficiency of electrical devices. It helps ensure that electrical systems are properly designed and that energy is used efficiently.
- Relation to Apparent and Reactive Power: In addition to active power, electrical systems also involve apparent power (the combination of active and reactive power) and reactive power (power associated with the phase difference between voltage and current). Active power is the portion of apparent power that performs useful work, while reactive power is required for the establishment of electromagnetic fields and voltage control in the system.
In summary, active power represents the real, usable power consumed by electrical devices and systems to perform work. It is a fundamental parameter in electrical engineering and plays a key role in power system analysis, energy management, and billing for electrical consumption.
What is an IC card?
An IC card, short for Integrated Circuit card, is a type of smart card that contains an embedded integrated circuit chip. This chip can store and process data, making IC cards highly versatile for various applications. IC cards are also commonly known as chip cards or smart cards. They are used in a wide range of fields for security, identification, and data storage purposes.
Key characteristics and uses of IC cards include:
- Embedded Chip: The most distinguishing feature of an IC card is the small integrated circuit chip embedded within it. This chip can perform various functions, such as data storage, data processing, encryption, and communication.
- Data Storage: IC cards can store a wide range of data, including personal information, access credentials, financial data, and more. The chip’s memory can be used to hold both static and dynamic data.
- Security: IC cards are known for their security features. The embedded chip can support cryptographic functions, making it difficult for unauthorized users to access or manipulate the card’s data.
- Identification: IC cards are commonly used for personal identification and authentication. They are used in access control systems, identity verification, and secure logins to systems and networks.
- Contact and Contactless Variants: IC cards come in two main variants: contact and contactless cards.
- Contact IC Cards: These cards require physical contact with a card reader for data transfer and communication. Common examples include EMV (Europay, Mastercard, and Visa) payment cards and some government-issued identification cards.
- Contactless IC Cards: These cards use radio-frequency identification (RFID) or near-field communication (NFC) technology to communicate wirelessly with card readers. They are commonly used for public transportation payment systems (e.g., contactless transit cards) and access control.
- Applications: IC cards have a wide range of applications, including:
- Payment Cards: Debit and credit cards with EMV chips for secure transactions.
- Identification Cards: Government-issued ID cards, driver’s licenses, and passports.
- Access Control: Cards used for access to buildings, secure areas, and computer networks.
- Transportation Cards: Cards used for fare payment on public transportation systems.
- Healthcare: Health insurance cards and patient identification cards.
- ** Loyalty and Membership Cards:** Cards used by retailers and organizations to track customer loyalty and memberships.
- SIM Cards: Subscriber Identity Module cards used in mobile phones.
- Durability: IC cards are designed to be durable and resistant to wear and tear, making them suitable for long-term use.
IC cards have become an integral part of modern life, offering convenience, security, and versatility in various industries. Their ability to securely store and process data makes them invaluable for applications where authentication, access control, and data protection are essential.
What are the main benefits of the transformation of the communication network?
The transformation of communication networks, often driven by advancements in technology, brings about numerous benefits that impact individuals, businesses, and society as a whole. These benefits contribute to improved connectivity, efficiency, and innovation across various sectors. Here are some of the main benefits of the transformation of communication networks:
- Enhanced Connectivity:
- Wider Geographic Coverage: Modern communication networks expand coverage to reach more remote and underserved areas, connecting people who were previously isolated.
- High-Speed Internet: High-speed broadband and 5G networks offer faster and more reliable internet access, enabling seamless online activities, including streaming, video conferencing, and cloud computing.
- Improved Communication:
- Real-Time Communication: Advanced networks enable real-time communication through voice and video calls, instant messaging, and social media, facilitating global connectivity.
- Rich Media Sharing: Networks support the sharing of multimedia content, such as photos, videos, and documents, enhancing communication experiences.
- Business and Economic Growth:
- Digital Transformation: Businesses can leverage modern communication networks to undergo digital transformation, enabling them to be more competitive, agile, and efficient.
- E-commerce: Robust online communication networks support the growth of e-commerce, allowing businesses to reach a global customer base.
- Education and Training:
- Online Learning: Communication networks enable online education and training, making learning accessible to people of all ages and backgrounds.
- Remote Collaboration: Students and professionals can collaborate on projects and research across geographical boundaries.
- Healthcare Services:
- Telemedicine: Communication networks facilitate remote healthcare consultations and monitoring, improving access to medical services, especially in rural areas.
- Health Data Sharing: Healthcare providers can securely share patient data for better diagnosis and treatment.
- IoT (Internet of Things):
- Smart Devices: Networks enable the proliferation of smart devices and IoT applications that enhance automation, convenience, and resource efficiency.
- Industrial IoT (IIoT): Industries can use IoT for predictive maintenance, process optimization, and cost savings.
- Smart Cities and Infrastructure:
- Efficient Urban Planning: Communication networks support smart city initiatives, leading to improved transportation, energy efficiency, and public services.
- Infrastructure Monitoring: Networks enable real-time monitoring of critical infrastructure, enhancing safety and security.
- Environmental Impact:
- Reduced Travel: Virtual meetings and remote work made possible by communication networks contribute to reduced carbon emissions by decreasing the need for physical commuting.
- Energy Efficiency: Smart grids and energy management systems powered by communication networks help reduce energy consumption.
- Emergency Response and Public Safety:
- Faster Response Times: Networks support quicker emergency response through improved communication and location-based services.
- Disaster Management: Networks aid in disaster preparedness, response, and recovery efforts.
- Global Collaboration:
- Cross-Border Partnerships: Communication networks foster international cooperation, research collaboration, and cultural exchange.
- Innovation Ecosystems: Innovation and knowledge sharing are accelerated through global collaboration.
- Data Analytics and Insights:
- Data Collection: Communication networks enable the collection of vast amounts of data, which can be analyzed for insights and decision-making.
- Big Data Applications: Businesses and governments use data analytics to improve services, personalize offerings, and optimize operations.
- Accessibility and Inclusivity:
- Access for All: Communication networks help bridge the digital divide by providing access to information and services for individuals with disabilities and those in underserved communities.
In summary, the transformation of communication networks offers a wide array of benefits that touch nearly every aspect of modern life. These benefits include improved connectivity, economic growth, enhanced communication, and advancements in fields such as healthcare, education, and environmental sustainability. They contribute to a more connected, efficient, and innovative world.
What are the applications of the GPRS module?
General Packet Radio Service (GPRS) modules are communication devices that provide wireless data connectivity to various applications. GPRS is a mobile data service that enables devices to transmit and receive data over cellular networks. GPRS modules are versatile and can be used in a wide range of applications. Here are some common applications of GPRS modules:
- Remote Monitoring and Control:
- GPRS modules are used in remote monitoring systems for various purposes, such as monitoring environmental conditions (temperature, humidity), industrial equipment, and utility meters.
- They enable remote control and automation of devices, such as turning lights on/off or opening/closing valves.
- Fleet Tracking and Management:
- GPRS modules are crucial in vehicle tracking and fleet management systems. They provide real-time location data, vehicle diagnostics, and route optimization.
- These modules can be used to monitor driver behavior, reduce fuel consumption, and improve overall operational efficiency.
- Security and Surveillance:
- GPRS-enabled security systems allow remote monitoring of homes, businesses, and assets.
- Surveillance cameras equipped with GPRS modules can transmit live video feeds and alert notifications to security personnel or property owners.
- Environmental Monitoring:
- GPRS modules are used in environmental monitoring systems to collect and transmit data from remote sensors. This includes monitoring air quality, water quality, and weather conditions.
- Researchers and government agencies use GPRS for collecting environmental data over a wide area.
- Smart Agriculture:
- GPRS modules help farmers and agricultural professionals monitor and manage crops, livestock, and irrigation systems.
- They enable remote control of irrigation pumps, soil moisture sensors, and weather stations.
- Asset Tracking:
- GPRS modules are used for tracking valuable assets, such as shipping containers, construction equipment, and high-value products during transportation.
- They provide real-time location updates and theft prevention.
- Point-of-Sale (POS) Systems:
- GPRS-enabled POS terminals are used by mobile vendors, food trucks, and businesses in locations without fixed internet connections.
- They allow for card payments and transaction processing on the go.
- Smart Energy:
- GPRS modules are integrated into smart meters for electricity, gas, and water utilities. They enable remote meter reading and data transmission.
- Consumers can monitor their energy consumption and make informed decisions about energy usage.
- Healthcare and Telemedicine:
- GPRS modules play a role in remote patient monitoring systems. They transmit health data from medical devices to healthcare providers.
- Telemedicine services use GPRS for real-time video consultations and patient data transmission.
- Telematics and Navigation:
- GPRS is used in vehicle navigation systems, providing real-time traffic updates, location-based services, and route planning.
- Ride-sharing and taxi services rely on GPRS for communication between drivers and passengers.
- IoT Applications:
- GPRS modules are integral to various Internet of Things (IoT) applications, connecting a wide range of devices and sensors to the internet.
- These applications span industries such as agriculture, healthcare, logistics, and smart cities.
- Emergency Services:
- GPRS modules can be used in emergency alert systems, helping authorities communicate important information to the public during disasters or emergencies.
These are just some of the many applications of GPRS modules. Their versatility and ability to provide wireless data connectivity make them valuable components in modern IoT, communication, and monitoring systems.
COMMENTS