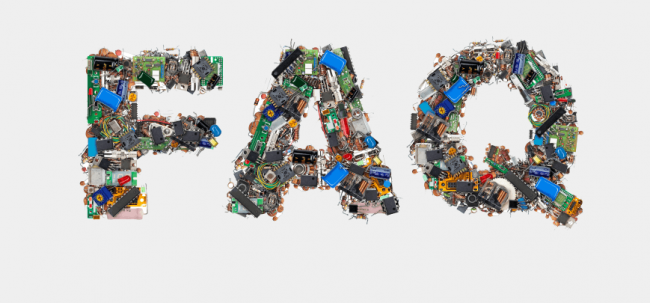
What is a central collision sensor?
A central collision sensor, also known as a center collision sensor, is a type of automotive safety technology designed to detect and respond to potential collisions occurring in the center of the vehicle. It is typically installed in the front or rear of the vehicle and uses various sensors, such as radar or cameras, to monitor the surrounding environment.
The central collision sensor continuously scans the area in front or behind the vehicle to detect the presence and proximity of objects, vehicles, or pedestrians. It analyzes the data received from the sensors to determine the risk of a collision and can activate various safety systems to mitigate or avoid the impact.
When a potential collision is detected, the central collision sensor can trigger warning signals to alert the driver, such as visual or auditory alerts. Additionally, it can activate pre-crash systems, such as automatic emergency braking, collision avoidance systems, or adaptive cruise control, to assist the driver in preventing or minimizing the severity of a collision.
The main purpose of a central collision sensor is to enhance vehicle safety by providing an additional layer of protection against frontal or rear collisions. By constantly monitoring the surroundings and providing timely warnings and interventions, it helps improve driver awareness and responsiveness, reducing the risk of accidents and mitigating their consequences.
What is the branch current method?
The branch current method, also known as the mesh current method or loop current method, is a technique used in electrical circuit analysis to determine the unknown currents flowing through different branches of a circuit. It is based on Kirchhoff’s current law (KCL), which states that the sum of currents entering a node in a circuit is equal to the sum of currents leaving that node.
The branch current method involves the following steps:
- Identify the branches: Identify and label all the branches in the circuit. A branch is a path between two nodes where current can flow.
- Assign variables: Assign variables (usually represented by letters) to the currents flowing through each branch. These variables are typically assigned in the direction of assumed current flow.
- Apply KCL: Apply Kirchhoff’s current law at each node in the circuit to form a set of simultaneous equations. Each equation represents the sum of currents entering and leaving a node and is expressed in terms of the assigned branch currents.
- Solve the equations: Solve the set of simultaneous equations obtained from step 3. This can be done using various techniques, such as substitution, elimination, or matrix methods.
- Determine branch currents: Once the equations are solved, the values of the assigned variables represent the branch currents in the circuit.
The branch current method is particularly useful for analyzing complex circuits with multiple current sources and interconnected branches. By assigning variables and applying KCL at each node, the method provides a systematic approach to determine the currents flowing through individual branches. This information can be used to calculate voltages, power dissipation, and other electrical characteristics of the circuit.
What is the detection method of the switch contact throttle position sensor?
The detection method of the switch contact throttle position sensor involves monitoring the position of the throttle valve in an internal combustion engine to provide feedback on the throttle opening to the engine control unit (ECU). The switch contact throttle position sensor is typically used in older throttle systems that utilize mechanical linkages.
The sensor consists of a variable resistor or potentiometer connected to the throttle shaft. As the throttle valve opens or closes, the resistance of the sensor changes accordingly. The detection method involves measuring this resistance and translating it into throttle position information.
Here are the steps involved in the detection method:
- Sensor Configuration: The switch contact throttle position sensor is typically designed with three terminals. Two terminals are connected to the ends of the resistor track, and the third terminal is connected to the wiper or contact arm that moves along the resistor.
- Voltage Application: A constant voltage is applied across the two end terminals of the sensor.
- Throttle Movement: As the throttle valve opens or closes, the mechanical linkage connected to the sensor causes the wiper or contact arm to move along the resistor track.
- Resistance Measurement: The ECU measures the voltage at the wiper or contact arm terminal, which corresponds to the resistance value of the sensor. This measurement is used to determine the position of the throttle valve.
- Throttle Position Calculation: The ECU compares the measured resistance value with predetermined values or a calibration curve to calculate the throttle position. The throttle position is usually expressed as a percentage, representing the opening or closing of the throttle valve.
- Feedback to ECU: The calculated throttle position information is then used by the ECU to adjust the fuel injection, ignition timing, and other engine parameters for optimal performance and emissions control.
What are the two special features of Rl4 in terms of structure?
Rl4, also known as ribosome-like 4, is an RNA molecule that plays a role in protein synthesis. It is a component of the large subunit of the ribosome and is involved in the peptidyl transferase reaction. While Rl4 does not possess any unique or special structural features, there are two notable aspects related to its structure:
- Conserved Structural Elements: Rl4 contains conserved structural elements that are crucial for its function. These elements include regions of secondary structure, such as stem-loops and helices, which are important for stabilizing the overall structure of the molecule. The conservation of these structural elements across different organisms reflects the significance of Rl4 in the ribosome’s function.
- Interaction with Ribosomal Components: Rl4 interacts with other components of the ribosome, including proteins and other RNA molecules, to form the functional ribosome complex. It participates in intricate molecular interactions within the ribosome, contributing to the overall stability and proper functioning of the ribosome during protein synthesis.
While the specific details of Rl4’s structure and its precise role in the ribosome’s function may vary depending on the organism, these two features of conserved structural elements and interactions with other ribosomal components are generally observed in Rl4 across different species.
What are the advantages of noninvasive detecting sensors?
Noninvasive detecting sensors offer several advantages compared to invasive or intrusive sensors. Some of the key advantages are:
- Safety: Noninvasive sensors do not require penetration or insertion into the body or the object being measured. This eliminates the risk of injury, infection, or tissue damage associated with invasive procedures. Noninvasive sensors are particularly beneficial in healthcare applications where patient safety is a primary concern.
- Comfort: Noninvasive sensors provide a more comfortable experience for the user or patient. They eliminate the discomfort, pain, or inconvenience associated with invasive methods, such as needle pricks or surgical procedures. This is especially important for long-term monitoring or repeated measurements.
- Convenience: Noninvasive sensors are generally easier to use and require minimal preparation or setup. They can be quickly applied or positioned on the body or the object being monitored, reducing the time and effort required for measurements. This convenience makes them suitable for various applications, including personal health monitoring, sports performance tracking, and industrial process monitoring.
- Reduced Risk of Infection: Invasive sensors carry the risk of introducing pathogens or causing infections at the site of insertion. Noninvasive sensors eliminate this risk, as they do not breach the skin or penetrate the body. This is especially crucial in medical settings where infection control is essential.
- Non-Destructive: Noninvasive sensors allow for measurements without altering or damaging the object or the sample being tested. This is valuable in applications where the integrity or preservation of the object is important, such as art restoration, material analysis, or non-destructive testing in industrial settings.
- Cost-Effective: Noninvasive sensors generally have lower overall costs compared to invasive methods. They eliminate the need for specialized equipment, sterilization procedures, or disposable components associated with invasive techniques. Noninvasive sensors can be reusable or have a longer lifespan, reducing the recurring expenses for replacements.
- Wide Range of Applications: Noninvasive sensors can be applied in a wide range of fields, including healthcare, sports, environmental monitoring, and industrial processes. They enable continuous or frequent measurements without causing discomfort or inconvenience to the individuals involved.
What are the main features of ZigBee technology?
ZigBee technology is a wireless communication protocol designed for low-power, low-data-rate applications. It offers several key features that make it suitable for a wide range of applications:
- Low Power Consumption: ZigBee devices are designed to operate on low power, making them ideal for battery-powered or energy-efficient applications. The protocol utilizes a sleep mode, where devices can conserve power by remaining in a dormant state until they need to transmit or receive data.
- Mesh Networking: ZigBee forms a mesh network, where devices can act as routers to relay data between other devices in the network. This feature enables the creation of large-scale networks with extended coverage and improved reliability. Mesh networking also provides self-healing capabilities, where devices can find alternate paths in case of network disruptions or device failures.
- Low Data Rate: ZigBee is optimized for low-data-rate applications, typically operating in the range of 20 to 250 kbps. This makes it suitable for applications that require intermittent or periodic data transfer, such as home automation, industrial monitoring, and sensor networks.
- Short Range Communication: ZigBee operates in the 2.4 GHz industrial, scientific, and medical (ISM) frequency band, allowing for short-range communication. The range typically extends up to 10-100 meters, depending on the environment and the power output of the devices. This range limitation ensures low interference and makes ZigBee suitable for applications within a confined space.
- Robustness and Reliability: ZigBee incorporates various mechanisms to ensure robust and reliable communication. It utilizes frequency hopping spread spectrum (FHSS) to avoid interference from other wireless devices operating in the same frequency band. It also employs error detection and correction techniques to enhance data integrity and reliability.
- Security: ZigBee provides built-in security features to protect data transmission and ensure network integrity. It supports various encryption methods, including symmetric key encryption and authentication mechanisms, to prevent unauthorized access and ensure secure communication within the network.
- Scalability: ZigBee networks can be easily expanded or modified to accommodate additional devices. New devices can be added to the network without significant disruption, and the mesh network self-configures to accommodate the new devices while maintaining network connectivity.
What are the main threats to cybersecurity?
Cybersecurity faces numerous threats that can compromise the confidentiality, integrity, and availability of digital systems and data. Some of the main threats include:
- Malware: Malicious software, such as viruses, worms, ransomware, and spyware, can infect systems and cause significant damage. Malware can steal sensitive information, disrupt operations, or provide unauthorized access to systems.
- Phishing and Social Engineering: Phishing involves fraudulent attempts to obtain sensitive information, such as passwords or financial data, by impersonating trusted entities through emails, messages, or websites. Social engineering techniques exploit human vulnerabilities to manipulate individuals into revealing confidential information or performing harmful actions.
- Data Breaches: Data breaches occur when unauthorized individuals gain access to sensitive data stored in databases or systems. This can lead to the exposure of personal information, financial loss, identity theft, or reputational damage.
- Denial-of-Service (DoS) and Distributed Denial-of-Service (DDoS) Attacks: DoS and DDoS attacks overwhelm a system, network, or website with a flood of traffic, rendering it inaccessible to legitimate users. These attacks disrupt services, cause financial losses, and can be used as a smokescreen for other malicious activities.
- Insider Threats: Insider threats involve individuals within an organization who misuse their authorized access to compromise data or systems. This can be intentional, such as malicious insiders seeking financial gain or revenge, or unintentional, such as employees falling victim to social engineering attacks.
- Advanced Persistent Threats (APTs): APTs are sophisticated, long-term cyber attacks typically carried out by well-funded and highly skilled attackers. APTs involve targeted infiltration, persistent monitoring, and stealthy exfiltration of data over an extended period. APTs are often difficult to detect and can cause significant damage to organizations.
- Ransomware: Ransomware is a type of malware that encrypts files or locks systems, demanding a ransom from the victim to restore access. Ransomware attacks can disrupt operations, lead to data loss, and result in financial losses if the ransom is paid.
- Internet of Things (IoT) Vulnerabilities: With the proliferation of connected devices, vulnerabilities in IoT systems pose a significant cybersecurity risk. Insecure IoT devices can be compromised to gain unauthorized access to networks, compromise data, or launch attacks.
- Zero-day Exploits: Zero-day exploits target vulnerabilities in software or hardware that are unknown to the vendor or have not yet been patched. Attackers exploit these vulnerabilities before a fix is available, making them challenging to detect and defend against.
- Supply Chain Attacks: Supply chain attacks target the software or hardware supply chain to infiltrate systems. Attackers compromise trusted suppliers or distributors to inject malicious code or hardware, which can then be distributed to unsuspecting users.
What should I pay attention to when conducting wireless environment testing?
When conducting wireless environment testing, there are several important factors to consider. Here are some key points to pay attention to:
- Test Objectives: Clearly define the objectives of your wireless environment testing. Determine the specific aspects you want to evaluate, such as signal strength, coverage, interference, data throughput, or network performance. This will guide your testing approach and help you focus on relevant parameters.
- Test Equipment: Select appropriate test equipment for your specific testing needs. This may include spectrum analyzers, signal generators, wireless network analyzers, or specialized testing tools. Ensure that the equipment is calibrated and properly configured for accurate measurements.
- Test Locations: Choose representative test locations that cover different areas of interest. Consider factors such as building layout, walls, obstacles, distance from access points, and areas with potential interference sources. Test both indoor and outdoor environments if applicable.
- Test Scenarios: Define various test scenarios that simulate real-world conditions. This may involve testing different configurations, client densities, traffic loads, or mobility scenarios. Create scenarios that reflect the typical usage patterns and environments in which the wireless network will operate.
- Signal Strength and Coverage: Measure the signal strength and coverage throughout the test locations. Use tools like signal meters or wireless network analyzers to assess the signal quality and identify areas with weak or no signal coverage. Pay attention to areas where coverage may be critical, such as office spaces, meeting rooms, or outdoor areas.
- Interference Analysis: Identify potential sources of interference in the wireless environment. Scan for other wireless networks, neighboring devices, or electronic equipment that may generate electromagnetic interference. Analyze the impact of interference on the wireless network performance and take appropriate mitigation measures if necessary.
What is cluster computing?
Cluster computing refers to the use of multiple interconnected computers or servers, called nodes, working together as a single system to perform computational tasks. In a cluster, each node operates independently but collaborates with other nodes to achieve a common goal. The nodes are typically connected through a high-speed network and share resources such as processing power, memory, storage, and input/output capabilities.
Clusters are designed to provide increased computing power, improved performance, scalability, and fault tolerance compared to individual standalone computers. By distributing computational tasks across multiple nodes, cluster computing allows for parallel processing, where different nodes work on different parts of a problem simultaneously, resulting in faster execution and increased efficiency.
What are the integrated sensitive units?
The integrated sensitive unit includes a resilient sensing element and a transducer.
COMMENTS